Antimicrobial drug resistance and the rapid emergence of resistant bacterial species continue to be a growing concern as it threatens the effectivity of traditional antimicrobial compounds against its target pathogens [1,2]. This evolutionary arms race demands the identification and development of novel antimicrobial compounds that have the potential to substitute antibiotic use and other similar applications. Bacteriocins, which are ribosomal-synthesized peptides produced by bacteria to inhibit the growth of closely related strains [2,3], are potential alternatives to traditional antibiotics [4]. Enterococcus faecalis CAUM157, a Gram-positive, facultatively anaerobic, non-spore-forming cocci [5], was observed to produce an antimicrobial protein with potent activity against a wide range of bacteria including periodontal disease-causing pathogens, Prevotella intermedia and Fusobacterium nucleatum [6], and Listeria monocytogenes, and Staphylococcus aureus (unpublished data). Whole-genome sequencing of strain CAUM157 was performed to further investigate the molecular characteristics of the bacteriocin gene structure.
E. faecalis CAUM157 was isolated from raw cow’s milk obtained from a local farm in Anseong, Korea. Strain CAUM157 was routinely grown in de Man, Rogosa, and Sharpe (MRS, Difco Laboratory, USA) broth supplemented with 1% L-cysteine at 37°C. The genomic DNA was extracted from 12 h cultures using QIAamp PowerFecal DNA Kit (Qiagen, Germany) according to the manufacturer’s instructions. Genomic DNA of strain CAUM157 was sent to ChunLab (Korea) and sequenced using the Pacific Biosciences (PacBio, USA) RSII Single Molecule Real-Time (SMRT) platform with 20 kb SMRTbell™ template library. The PacBio reads were assembled de novo using the PacBio SMAR Analysis ver. 2.3.0 program. Genome annotation was performed with the Rapid Annotation using Subsystem Technology (RAST) using default parameters [7] and CLgenomics™ ver. 1.55 software. Transfer RNAs (tRNAs) were identified using tRNAscan-SE ver. 1.3.1 [8]. Ribosomal RNAs (rRNAs) and non-coding RNAs were identified using INFERNAL ver. 1.1.3 software with Rfam 12.0 database [9]. Functional annotation of protein-coding sequences (CDSs) was performed using the PRODIGAL ver. 2.6.2 software [10] and compared to protein databases (SwissProt, KEGG, SEED, EggNOG) using USEARCH ver. 8.1 [11]. The complete genome of E. faecalis CAUM157 (Fig. 1) has a length of 2,972,812 bp with a G+C content of 37.44% assembled into 2 contigs with an N50 value of 2,913,602 bp. The genome contains 2,830 coding genes, 12 rRNAs, and 61 tRNAs (Table 1).
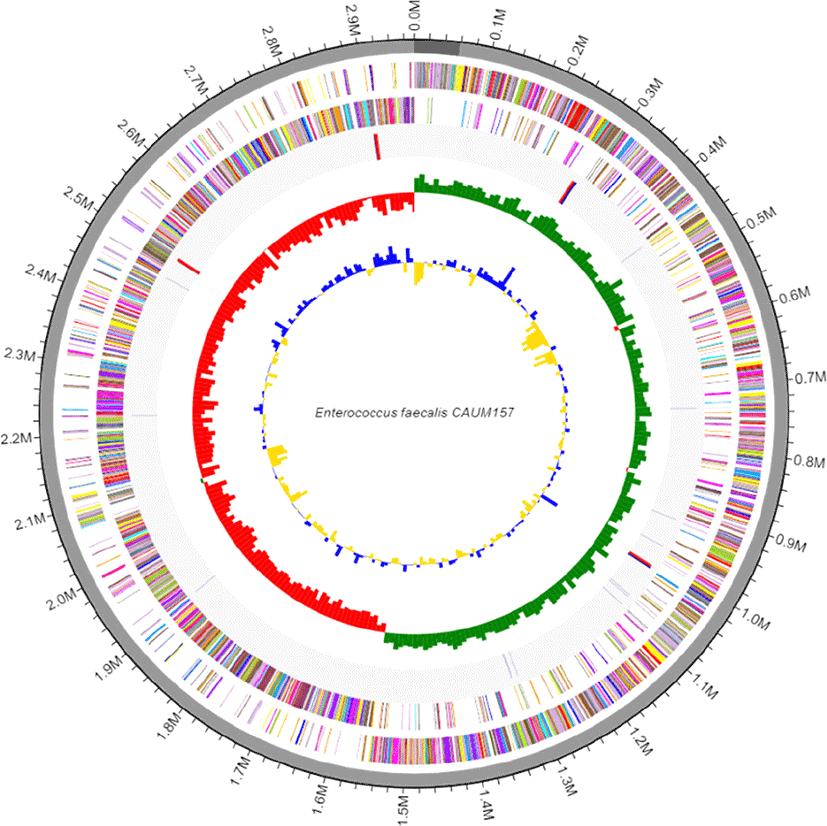
Attribute | Value |
---|---|
Genome size (bp) | 2,972,812 |
GC content (%) | 37.4 |
No. of contigs | 2 |
Total genes | 2,903 |
Protein-coding gene | 2,830 |
tRNA | 61 |
rRNA | 12 |
Plasmids | 0 |
GenBank accession no. | JACSYK000000000 |
Putative bacteriocin-encoding gene clusters were determined in silico using the BAGEL4 software tool [12] which revealed 1 area of interest (AOI) in contig CM157.00001, corresponding to the bacteriocin genes. Two open reading frames (ORFs) encoding the core peptides of bacteriocin MR10A (E value, 2×10–27) and MR10B (E value, 1×10–27) were detected. The amino acid sequences of the two core peptides deduced from the putative bacteriocin gene share a similar sequence and lack the YGNGVXC motif characteristic of the leader sequence, which suggests that the bacteriocin is of class IIB – leaderless two-peptide bacteriocin [13]. Additionally, the amino acid sequence exhibited a high degree of similarity with a previously described bacteriocin (MR10A and MR10B) from E. faecalis [14]. The corresponding sequences are homologous with the plasmid-encoded enterocins L50A and L50B described in E. faecium L50 [15] except for a conservative change (Glu 38 to Asp) in MR10A and two residue change (Thr 9 to Ala, and Leu 15 to Phe) in MR10B [16]. Furthermore, genes encoding for self-immunity and ABC-transport system (efflux RND transporter, ABC transporter ATP-binding protein, and ABC transporter permease) were detected downstream of the putative bacteriocin genes.