The genus Chryseobacterium is composed of Gram-negative, aerobic, non-fermentative, psychrotrophic bacilli with characteristic yellow pigmentation. The genus is considered a part of the normal environmental microflora and has been associated with human infections [1,2]. Recently, several novel species have been isolated from raw milk samples, including C. mulctrae KACC 21234T [3-6]. And although Chryseobacterium is not part of the normal microflora of milk, contamination can occur during the milking process (i.e., from cow udder, human handling, and immediate environment) or transport and processing [7]. Psychrotrophs can take advantage of the high nutritional content of milk and grow at low temperatures (7°C and below). The activity of these microorganisms may lead to different types of milk spoilage – souring, gas production, proteolysis, ropiness, change in milk fat, flavor defect, and color defect. In addition, some contaminating microorganisms are potentially pathogenic [8].
The presence of lipolytic and proteolytic microorganisms in milk may lead to a variety of defects and shortened shelf-life through the production of extracellular enzymes that can hydrolyze proteins (b-casein and as-casein) and triglycerides, ultimately causing spoilage of the product. Proteases, particularly plasmin, are linked with gelation of UHT sterilized milk, development of bitterness in milk, and reduction in yield of soft cheese while the action of lipases affects the flavor profile of dairy products [8]. Moreover, the extracellular enzymes synthesized by psychrotrophic bacteria are heat stable, withstanding pasteurization conditions (72°C for 15 sec) and even ultrahigh temperature (UHT, 132°C for 2 sec) processes, presenting a challenge in securing the quality and safety of milk and dairy products [8,9].
C. mulctrae KACC 21234T was isolated based on its proteolytic activity on skim milk agar plate (SMA; 5% skim milk), incubated at 10°C for 10 days. Strain KACC 21234T was routinely cultured in tryptic soy agar (TSA, BD Difco, USA) at 30°C [6]. The genomic DNA was extracted using QIAamp PowerFecal DNA kit (Qiagen, Germany) and sent to ChunLab (Korea) for sequencing using PacBio RSII Single Molecule Real-Time (SMRT) platform with 20 kb SMRTbellTM template library. De novo assembly of the PacBio reads was performed using the PacBio SMRT analysis software ver. 2.3.0.
Genome annotation was perdformed using the Rapid Annotation using Subsystem Technology (RAST) using default parameters [10]. Transfer RNAs and ribosomal RNAs were identified using tRNAscan-SE ver. 1.3.1 [11] and INFERNAL ver. 1.1.3 software using the Rfam 12.0 database [12], respectively (Fig. 1, 2). The genome features of C. mulctrae KACC 21234T are listed in Table 1.
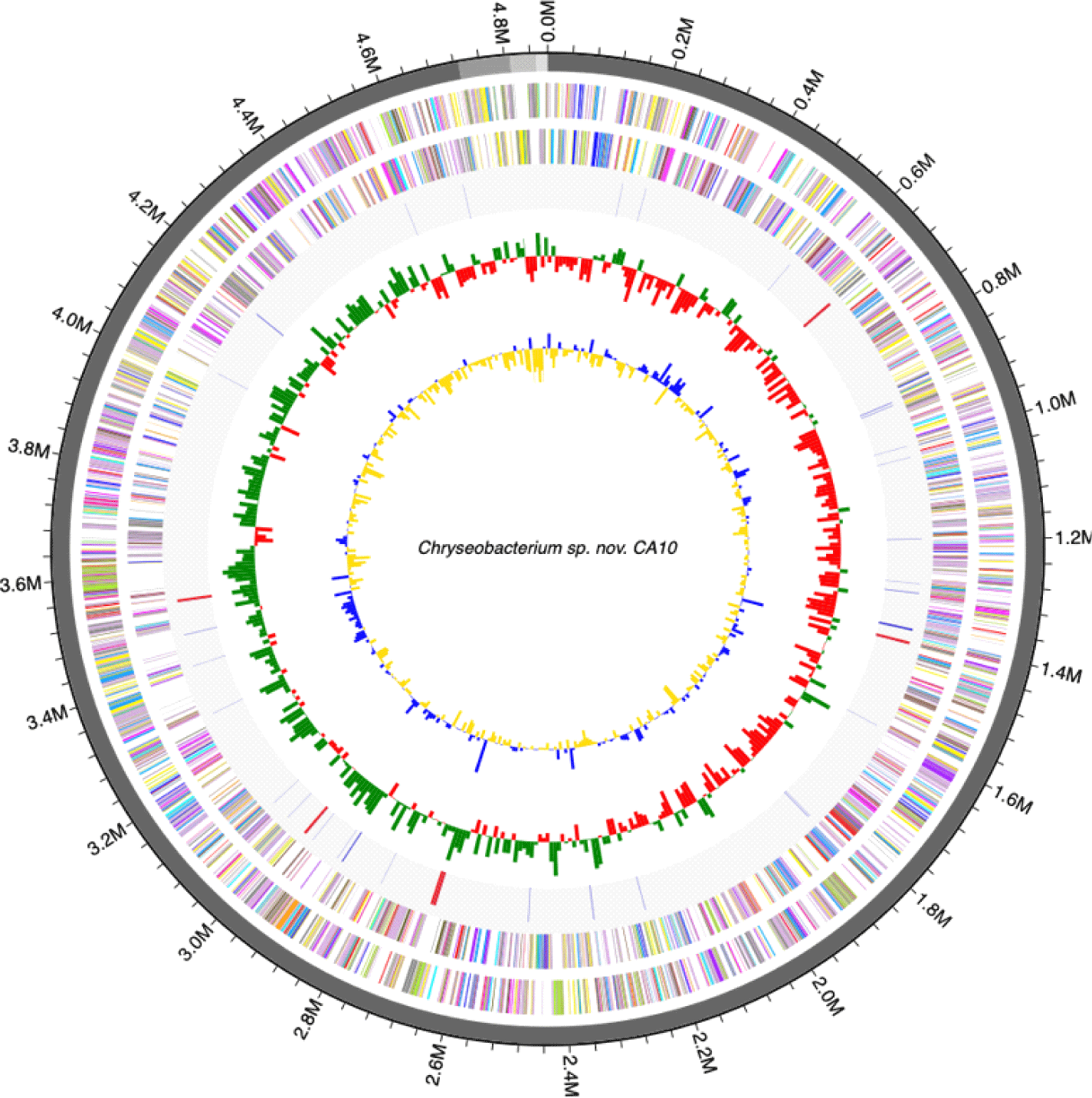
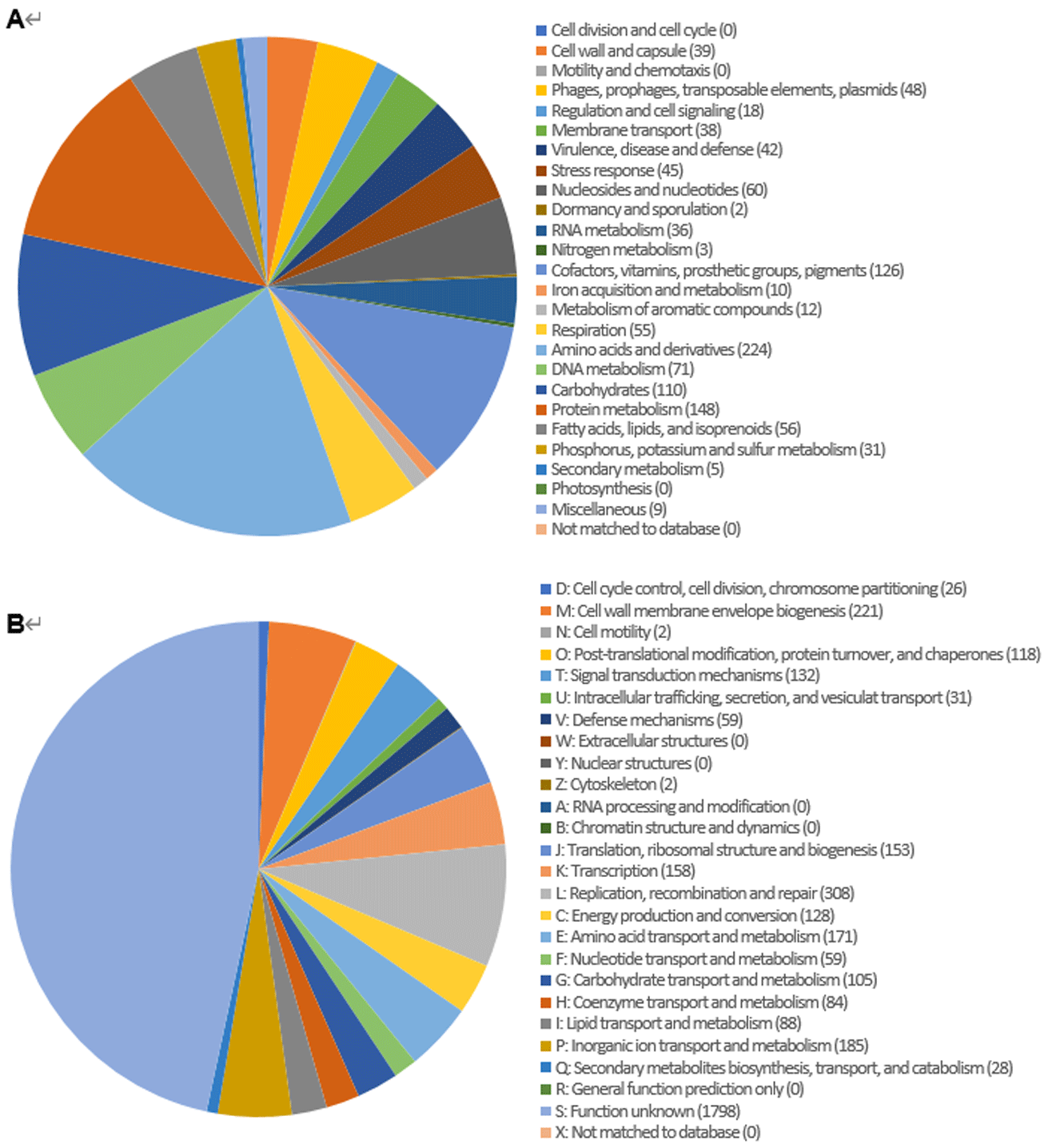
Attribute | Value |
---|---|
Genome size (bp) | 4,868,651 |
G+C content (%) | 33.8 |
No. of contigs | 4 |
Protein-coding genes | 4,610 |
tRNA | 75 |
rRNA | 18 |
Plasmids | 0 |
GenBank Accession No. | VAJL00000000 |
Functional genome annotation revealed several genes encoding for proteases and lipases (Table 2). Several studies have reported that members of Chryseobacterium showed greater spoilage ability than Pseudomonas spp. based on its proteolytic and lipolytic activity [7,13]. Additionally, although less frequently reported, the production of phospholipases (CS110_01857 and CS110_02528) was associated with sweet curdling and bitter cream in milk due to the aggregation of fat globules. C. mulctrae KACC 21234T also has genes for b-galactosidase (CS110_00343), which may cause unwanted hydrolysis of β-galactosidic bonds in lactose.
Gene name | Length (bp) | Product | Predicted function | Reference | |
---|---|---|---|---|---|
Protease | |||||
CS110_00311 | 1,581 | Carboxypeptidase | Aminopeptidase, metallodipeptidase | [14] | |
CS110_00621 | 687 | Endopeptidase Clp | Chymotrypsin-like activity | [15] | |
CS110_00616 | 2,133 | Oligopeptidase B | Serine-type endopeptidase, hydrolysis of carboxyl side of basic amino acids | [16] | |
CS110_01328 | 1,539 | Peptidyl-Asp metalloendopeptidase | Cleave Xaa-Cya or Xaa-Asp at N-terminus | [17] | |
CS110_02075 | 1,797 | Prolyl oligopeptidase | Selective cleavage of peptide bonds at the carboxyl group of internal proline residue | [18] | |
Lipase | |||||
CS110_01857 | 1,038 | Phospholipase A(2) | Cleaves fatty acid in position 2 of phospholipids | [19] | |
CS110_02528 | 528 | Phospholipase C | Lipid catabolism, phospholipolysis | [20] | |
CS110_03091 | 939 | Acylglycerol lipase | Hydrolysis of monoacylglycerol | [21] |
Antimicrobial resistance genes were also detected using the Resistance Gene Identifier with The Comprehensive Antibiotic Resistance Database (https://card.mcmaster.ca/analyze/rgi). Specifically, CPS-1, adeF, and qacG, which confers resistance to carbapenem, fluoroquinolone and tetracycline, and antiseptics, respectively, were identified. Furthermore, two open reading frames (ORF) encoding a putative bacteriocin (Linocin M18 and Carocin D) were identified via BAGEL4 (http://bagel4.molgenrug.nl/). However, there were no immunity and transport proteins associated with the bacteriocin genes.
The in-silico analyses of C. mulctrae KACC 21234T genome revealed the presence of various proteolytic and lipolytic enzymes, bacteriocins, and antimicrobial resistance genes which highlights the risks involved in microbial contamination of milk. Thus, it is imperative to develop effective screening methods for the detection of contaminating microorganisms and their enzymes to improve the quality and safety of milk and related products.